Clinical implications of three-dimensional mitral valve modelling, printing and simulation in mitral valve surgery
Introduction
The continuous trend towards less invasive procedures has increased the complexity of cardiac operations. Minimally invasive techniques require a different set of surgical skills as surgeons work through a smaller incision with long shafted instruments. Minimally invasive mitral valve surgery (MIMVS) has shown to be a safe and effective alternative compared to conventional sternotomy and has become widely accepted in many experienced centers (1,2). However, not all patients are suitable for a minimally invasive approach. More importantly, not all patients will benefit from a minimally invasive approach. Therefore, adequate patient selection and precise preoperative planning are essential for successful adaptation of minimally invasive techniques (3).
Modern imaging modalities are a mandatory component in patient selection and preoperative planning in cardiothoracic surgery. In mitral valve surgery, preoperative transoesophageal echocardiography (TOE) and computed tomography (CT) angiography increased preoperative knowledge on patient’s specific anatomy and pathology. Understanding the complex anatomy of the mitral valvular apparatus is essential for successful repair. Preoperative TOE provides anatomical insight to assess the feasibility of repair and CT is used to evaluate anatomical eligibility for a minimally invasive approach. The introduction of advanced reconstruction software improved the potential to create virtual 3D reconstructions, which can be rotated and segmented into layers. The reconstructions allow surgeons to enhance their understanding of the visuospatial relations between anatomical structures from a surgical view. Currently, virtual reconstructions are limited by being displayed on two-dimensional working stations, and not being displayed in real-time. In contrast, rapid prototyping, a technique used to generate prototype models, allows surgeons to develop a superior understanding of the anatomy and improved operative planning through the ability to interact directly with patient’s printed anatomy and pathology.
Since 1980, 3D printing has primarily been used in industrial design and manufacturing. With the introduction of more user friendly computer-aided design software, 3D printing made its way into the medical field (4). In addition, the cost of 3D printers and printing materials, in combination with the manufacturing time, have significantly decreased during the recent years, excluding the necessity of outsourcing by clinicians. Rapid prototyping has already shown to be a valuable tool in orthopaedics (5), neurosurgery (6) and plastic surgery (7). One of the major advantages of 3D printing is the capacity to directly translate a patient specific model into a tangible model. Nowadays, this technique has also emerged in the field of cardiovascular disease and cardiothoracic surgery (8).
Simultaneously with technical improvements in imaging modalities and patient specific modelling, preoperative simulation has gained increasing interest because of the limitations of the apprentice model. Arguments for the rationale for simulation based training include the European Working Time Directive (9), limiting the working hours of residents and their possibilities to develop their surgical skills intra-operatively (10), more technical procedures (minimally invasive surgery) with steep learning curves (11), operation room efficacy, ethical issues regarding patient safety (12) and a more complex, better informed and more demanding patient population. In the field of cardiac surgery, simulation-based training has been introduced in coronary bypass surgery for vascular anastomosis (13-15), aortic cannulation (16) and mitral valve surgery (17,18), ranging from low- to high-fidelity simulators. Simulation has the potential to reduce traditional learning curves and prevent trial-and-error in actual patients.
Patient-specific simulation, a combination of both 3D modelling and 3D printing, could serve as the ideal planning method in preparation of complex surgery.
In our center, a process for modelling and 3D printing of different mitral valve pathologies for procedural planning and simulation, based on 3D transoesophageal echocardiography, was developed. In this manuscript, we present the clinical implications of 3D modelling, printing and simulation in mitral valve surgery.
The process of rapid prototyping can roughly be divided into four major steps, namely data acquisition, data conversion, mesh refinement and rapid prototyping. Current 3D printing techniques do not allow high-quality printing in flexible, tissue-like materials for simulation purposes. Therefore, models specific for simulation of endoscopic mitral valve repair require 2 additional steps; negative mould fabrication and casting (Figure 1).
A patient-specific 3D model begins with clinical imaging. In our cases, imaging data were obtained throughout the entire cardiac cycle by 3D TOE using a Philips iE33 device with a 3D TOE probe (Philips Medical Systems, Andover, MA, USA). During data acquisition the mitral valve should be as close as possible to the probe, with minimization of movement to reduce artefacts. The ideal image acquisition possesses a completely dark blood pool with completely bright myocardium and mitral valvular tissue. The current standard ultrasound devices do not allow to directly export the data eligible for printing. Therefore, after a high-quality volumetric 3D image dataset is acquired, the data is converted to a cartesian Digital Imaging and Communications in Medicine (DICOM) format in QLAB 9.1 (Philips Medical Systems, Andover, MA, USA).
After export, the data is converted to a dynamically 3D reconstructed heart valve dataset using dedicated software (Vesalius3D, PS-Medtech, Amsterdam, Netherlands). Unfortunately, due to quality constrains, it is currently not possible to acquire, reconstruct and print the subvalvular apparatus. Therefore, only the mitral valve annulus and leaflets are reconstructed. In order to capture the coaptation defect itself, the mitral valve is reconstructed in the mid-systolic phase.
Next, the 3D reconstructed valve is exported into a Surface Tessellation Language (STL) file, which is the standard format in 3D printing. Mesh refinements are performed in MeshLab (Istituto di Scienza e Tecnologie dell’Informazione, Pisa, Italy) and afterwards manufactured into a model on a 1:1 scale. During this process, isolated and connected components with diameters smaller than 25% of the maximum diameter are deleted.
Printing is performed using selective laser stereolithography, which is considered the gold standard in 3D biomodel production. In cases where a patient-specific model was fabricated for our high-fidelity simulator two additional steps were required. In addition, for simulation-based training purposes deformable, tissue-mimicking silicone is used to allow suture placement. First, a three-piece negative mould was constructed, after which a tissue-like silicone valve could be casted. A more detailed process for modelling and three-dimensional printing has been described elsewhere (19).
Case presentation
The presented work flow for 3D reconstruction and printing can be used for a plethora of mitral valve pathologies and allowed us to reconstruct and print different mitral valve pathologies. We present 3 cases of clinical implication of mitral valve 3D printing.
Case 1: mitral valve repair for posterior leaflet prolapse and annular dilatation
A 63-year-old man with severe mitral valve regurgitation based on posterior leaflet prolapse and annular dilatation was found eligible for mitral valve surgery. Transthoracic echocardiography showed a normal right and left ventricular function with an end diastolic diameter of 58 millimetres. Transoesophageal echocardiography showed severe mitral valve regurgitation with an eccentric jet based on a P2 prolapse with chordal rupture. Also, a mild prolapse of the P1 segment was found. Echocardiography revealed no aortic insufficiency and a trace of tricuspid valve insufficiency. Computed tomography reconstruction revealed no contraindications for peripheral cannulation and the patient was accepted for minimally invasive mitral valve repair. A three-piece negative mould was created based on imaging acquisition with TOE (Figure 2). After casting the valve in silicone, the valve was mounted in the simulator for in vitro preoperative planning (Figure 3). Based on simulation, 2 pairs of neochordae were implanted in the P2 region. Additionally, 1 pair of neochordae was placed at the P1 region and 1 pair at the P3 region. To stabilize the repair, a semi-rigid annuloplasty ring (Medtronic, Dublin, Ireland), size 34, was implanted (Figure 2).
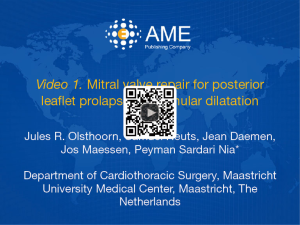
Case 2: mitral valve repair for posterior leaflet prolapse
A 64-year-old male with no medical history presented with increasing dyspnoea. A transthoracic echocardiography showed a left ventricular ejection fraction of 68%, left ventricle diastolic diameter of 52 mm, left ventricle systolic diameter of 32 mm and severe mitral regurgitation with an effective regurgitant orifice of 0.53 cm2. The mechanism of regurgitation was a prolapse of the P2 segment of the posterior leaflet. No other valve disease was observed. The patient was discussed in the mitral valve heart and found eligible for minimally invasive mitral valve surgery. A CT scan was performed, which showed no contraindications. A 3D negative mould and silicone casting was performed for preoperative simulation (Figure 4). Based on simulation, the preoperative strategy included 3 pair of neochordae and a stabilizing ring (Figure 5).
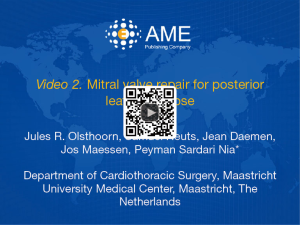
Case 3: transapical off-pump mitral valve repair
An 81-year-old female known with mitral valve regurgitation since 2017 presented at our outpatient clinic with progression of dyspnea. Transthoracic and transoesophageal showed severe MR based on posterior leaflet prolapse due to chordal rupture with a normal right and left ventricular function (Figure 6). Additional coronary angiography showed no coronary artery disease. The patient was discussed in our dedicated mitral valve heart team and was selected for transapical off-pump mitral valve repair using the NeoChord device based on an eligible patient anatomy, eligible valvular pathology and eligible ventricular dimensions in combination with her oncologic medical history and other comorbidities.
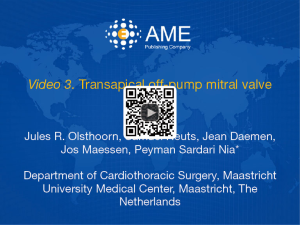
Based on TOE, an interactive 3D reconstruction was acquired (Figure 6). For transapical off-pump mitral valve repair, a rigid plastic prototype was directly printed using selective laser stereolithography (Figure 7) The patient-specific model was realized in the mid-systolic phase to assess the maximal coaptation defect. The coaptation defect allowed the surgical team to predict the probability of reduction of MR based on sufficient leaflet overlap. In cases with abundant overlap, sufficient tissue can be pulled into the ventricle to create of an adequate coaptation area. Finally, the model can be used intraoperatively to mark the location of the neochord.
Discussion
This report describes the clinical implication of 3D reconstructions, 3D printing and simulation in mitral valve surgery. We developed a standardized workflow for the creation of 3D printed mitral valves. With the help of our step-by-step workflow, it was feasible to create rigid plastic and silicone models of different mitral diseases. All reconstructions were based on 3D TOE, performed during preoperative work-up. The manufactured models assisted in selecting patients for the appropriate intervention by visualization of the exact valve pathology during multi-disciplinary heart team meetings. Additionally, the patient specific mitral valve prints helped to simulate the operation and determine the optimal intraoperative strategy.
Rapid prototyping has emerged in many subdomains in the medical field. Three-dimensional printing is one of the most promising techniques for preoperative planning and is expected to play an important role in personalized medicine in the future. Patient-specific models could subsequently be used to assist in decision making and procedural planning. Selecting the right patient for the right approach could help improve surgical and patient outcomes. As image acquisition is an important basic step for successful 3D printing, close collaboration between radiologist, echocardiologist and cardiothoracic surgeons is of utmost importance. The multidisciplinary valve heart team could be the basis for embankment of the different subdomains (23).
Transoesophageal echocardiography is the cornerstone for our preoperative 3D mitral valve modelling. Compared to other imaging modalities, TOE has the advantages of instant feedback and the ability to directly review the 3D image dataset. Additionally, there is no need for intravenous contrast or radiation exposure, as in computed tomography. Magnetic Resonance Imaging on the other hand, can be time consuming and more expensive. In our center, preoperative 3D TOE is performed by the same imaging cardiologists discussing the patient in the mitral valve heart team, guiding our transapical procedures intraoperatively and assessment of postoperative MR grading after weaning from bypass. Furthermore, other disciplines that use 3D echocardiographic acquisitions, like gynecologist and nephrologist may also benefit from these techniques, while applications are not limited to cardiac surgical procedures. Almost all software is freely available and has an intuitive interface.
Besides the role of rapid prototyping in the preoperative pathway, models are also helpful for training of surgeons and residents. Simulation with patient-specific models can shorten learning curves and ultimately determine intraoperative strategies and prevent intraoperative complications. Furthermore, these could even be used in the beginning of medical school to help students understand anatomy more easily.
Limitations
The accuracy of the 3D printed models used for patient care could raise concern. Current 3D printers can sufficiently print clinically relevant details with a margin of <1 mm. However, as these models are also dependent on post-processing, they are directly linked to operator interpretation and subjected to inter- and intra-observer variability. Standardization and automatization of the workflow is mandatory to make it more reproducible and generally accessible.
One of the major limitations of rapid prototyping is the workaround time. Currently, the average time for fabrication of rigid models, ranges from 4 hours to 1 day. For now, production of silicone models can even take up to 3 days from the image acquisition to the final silicone model. However, with the evolution of 3D printing, we foresee direct printing in silicone to be possible in the near future, shortening production times. Nowadays, the cost for our current models ranges from 15–40 euro for rigid printed models. The expenses for our silicone models, including their negative mould, are around €300, which can be subdivided into €210 for a single mould and €90 for casting.
The current report only shows a small sample of pathologies found in mitral valve surgery. We deliberately choose pathologies which are most common. However, one could argue that these are the most ‘simple’ repairs, which does not need extensive preoperative planning. The study was intended to give an insight in the possibilities of mitral valve modelling and 3D printing and not to compare the results of 3D printing head-to-head.
Conclusions
Three-dimensional mitral valve modelling and printing can enhance surgeon’s knowledge on patient specific anatomy and facilitate planning of complex surgery. Extensive preoperative planning with patient specific models may directly affect patient selection for the appropriate techniques. Furthermore, simulation based training with patient specific models can help surgeons and residents to gain proficiency in minimally invasive mitral valve surgery, and could ultimately shorten learning curves and improve patient outcomes.
Acknowledgments
Funding: None.
Footnote
Provenance and Peer Review: This article was commissioned by the editorial office, Journal of Visualized Surgery for the series “Minimally Invasive Mitral Valve Surgery”. The article has undergone external peer review.
Conflicts of Interest: The series “Minimally Invasive Mitral Valve Surgery” was commissioned by the editorial office without any funding or sponsorship. PSN served as the unpaid Guest Editor of the series and reports as Inventor of high-fidelity mitral valve simulator that is commercialized; Consultant for Neochord; Consult for Fujifilm cooperation; Consult for Edwards Lifescience. The authors have no other conflicts of interest to declare.
Ethical Statement: The authors are accountable for all aspects of the work in ensuring that questions related to the accuracy or integrity of any part of the work are appropriately investigated and resolved. All procedures performed in studies involving human participants were in accordance with the Helsinki Declaration (as revised in 2013). Written informed consent was obtained from the patient for publication of this manuscript and any accompanying images.
Open Access Statement: This is an Open Access article distributed in accordance with the Creative Commons Attribution-NonCommercial-NoDerivs 4.0 International License (CC BY-NC-ND 4.0), which permits the non-commercial replication and distribution of the article with the strict proviso that no changes or edits are made and the original work is properly cited (including links to both the formal publication through the relevant DOI and the license). See: https://creativecommons.org/licenses/by-nc-nd/4.0/.
References
- Modi P, Hassan A, Chitwood WR Jr. Minimally invasive mitral valve surgery: a systematic review and meta-analysis. Eur J Cardiothorac Surg 2008;34:943-52. [Crossref] [PubMed]
- Sundermann SH, Sromicki J, Rodriguez Cetina Biefer H, et al. Mitral valve surgery: right lateral minithoracotomy or sternotomy? A systematic review and meta-analysis. J Thorac Cardiovasc Surg 2014;148:1989-95.e4. [Crossref] [PubMed]
- Heuts S, Maessen JG, Sardari Nia P. Preoperative planning of left-sided valve surgery with 3D computed tomography reconstruction models: sternotomy or a minimally invasive approach? Interact Cardiovasc Thorac Surg 2016;22:587-93. [Crossref] [PubMed]
- Rengier F, Mehndiratta A, von Tengg-Kobligk H, et al. 3D printing based on imaging data: review of medical applications. Int J Comput Assist Radiol Surg 2010;5:335-41. [Crossref] [PubMed]
- Eltorai AE, Nguyen E, Daniels AH. Three-Dimensional Printing in Orthopedic Surgery. Orthopedics 2015;38:684-7. [Crossref] [PubMed]
- Randazzo M, Pisapia JM, Singh N, et al. 3D printing in neurosurgery: A systematic review. Surg Neurol Int 2016;7:S801-9. [Crossref] [PubMed]
- Chae MP, Rozen WM, McMenamin PG, et al. Emerging Applications of Bedside 3D Printing in Plastic Surgery. Front Surg 2015;2:25. [Crossref] [PubMed]
- Sardari Nia P, Heuts S, Daemen J, et al. Preoperative planning with three-dimensional reconstruction of patient's anatomy, rapid prototyping and simulation for endoscopic mitral valve repair. Interact Cardiovasc Thorac Surg 2017;24:163-8. [PubMed]
- Schimmack S, Hinz U, Wagner A, et al. Maximizing time from the constraining European Working Time Directive (EWTD): The Heidelberg New Working Time Model. Health Econ Rev 2014;4:14. [Crossref] [PubMed]
- Reznick RK, MacRae H. Teaching surgical skills--changes in the wind. N Engl J Med 2006;355:2664-9. [Crossref] [PubMed]
- Arora KS, Khan N, Abboudi H, et al. Learning curves for cardiothoracic and vascular surgical procedures--a systematic review. Postgrad Med 2015;127:202-14. [Crossref] [PubMed]
- Moore FD. Ethical problems special to surgery: surgical teaching, surgical innovation, and the surgeon in managed care. Arch Surg 2000;135:14-6. [Crossref] [PubMed]
- De Raet JM, Arroyo J, Buchner S, et al. How to build your own coronary anastomosis simulator from scratch. Interact Cardiovasc Thorac Surg 2013;16:772-6. [Crossref] [PubMed]
- Fann JI, Caffarelli AD, Georgette G, et al. Improvement in coronary anastomosis with cardiac surgery simulation. J Thorac Cardiovasc Surg 2008;136:1486-91. [Crossref] [PubMed]
- Fann JI, Calhoon JH, Carpenter AJ, et al. Simulation in coronary artery anastomosis early in cardiothoracic surgical residency training: the Boot Camp experience. J Thorac Cardiovasc Surg 2010;139:1275-81. [Crossref] [PubMed]
- Schiralli MP, Hicks GL, Angona RE, et al. An inexpensive cardiac bypass cannulation simulator: facing challenges of modern training. Ann Thorac Surg 2010;89:2056-7. [Crossref] [PubMed]
- Verberkmoes NJ, Verberkmoes-Broeders EM. A novel low-fidelity simulator for both mitral valve and tricuspid valve surgery: the surgical skills trainer for classic open and minimally invasive techniques. Interact Cardiovasc Thorac Surg 2013;16:97-101. [Crossref] [PubMed]
- Sardari Nia P, Daemen JHT, Maessen JG. Development of a high-fidelity minimally invasive mitral valve surgery simulator. J Thorac Cardiovasc Surg 2019;157:1567-74. [Crossref] [PubMed]
- Daemen JHT, Heuts S, Olsthoorn JR, et al. Mitral valve modelling and three-dimensional printing for planning and simulation of mitral valve repair. Eur J Cardiothorac Surg 2018; [Epub ahead of print]. [PubMed]
- Olsthoorn JR, Heuts S, Daemen J, et al. Mitral valve repair for posterior leaflet prolapse and annular dilatation. Asvide 2019;6:178. Available online: http://www.asvide.com/article/view/32377
- Olsthoorn JR, Heuts S, Daemen J, et al. Mitral valve repair for posterior leaflet prolapse. Asvide 2019;6:179. Available online: http://www.asvide.com/article/view/32378
- Olsthoorn JR, Heuts S, Daemen J, et al. Transapical off-pump mitral valve repair. Asvide 2019;6:180. Available online: http://www.asvide.com/article/view/32379
- Heuts S, Olsthoorn JR, Hermans SMM, et al. Multidisciplinary decision-making in mitral valve disease: the mitral valve heart team. Neth Heart J 2019;27:176-84. [Crossref] [PubMed]
Cite this article as: Olsthoorn JR, Heuts S, Daemen J, Maessen J, Sardari Nia P. Clinical implications of three-dimensional mitral valve modelling, printing and simulation in mitral valve surgery. J Vis Surg 2019;5:56.