Comprehensive evaluation of liver resection procedures: surgical mind development through cognitive task analysis
Introduction
Surgical planning is a prerequisite for safe and effective surgical practice. Surgical decision-making is a dynamic process and will not be completed until the end of the operation. Liver resection is a complex surgical procedure, which involves consideration of merged anatomical liver lobules, sufficiently intertwined intrahepatic vascular supply, and biliary trees (Glissonian pedicles); liver resection therefore demands advanced skills and expert surgical techniques (1,2). In surgical practice, skill development for laparoscopic liver surgery is substantially delayed compared with other surgical disciplines (1,3). In addition, surgical residents are less trained and prepared for liver resection (4). Resident training methods for liver surgery encounter many barriers (3,4). Unprepared surgical minds and unawareness of surgical planning are frequently observed in daily surgical education. Furthermore, surgical residents have limited work hours dedicated to training nowadays. Therefore, efficient learning methods are warranted.
Cognitive task analysis (CTA) is aimed at understanding tasks that require considerable mental activity from experts, e.g., decision-making, problem-solving, troubleshooting, identification of potential procedural errors, and judgment (5). Collective evidence on CTA as a surgical training method has revealed that CTA improves surgical outcome parameters, including operation time, surgical precision, and surgical accuracy; CTA results in an overall error reduction in both simulated and real-world environments (5). Through CTA, key steps and decision nodes that are omitted easily by experts during surgery can be identified and emphasized (6). Furthermore, video-based coaching is a complementary educational model to maximize the performance from every surgical exposure (7). The easily overlooked steps and decision nodes add up to seemingly “invisible” mind-sets that are crucial for surgical maturation and for mastery of liver resection through open or laparoscopic approaches. Therefore, the present study attempts to improve surgical training and coaching by providing an efficient learning module for liver resection through the application of modern educational technology.
Key elements of surgical planning for liver resection
Adequate, integrated, and flexible surgical planning is an important “invisible” skill that distinguishes experienced surgeons from trainee surgeons. The process of surgical planning can be based on (I) an established consensus or criteria [such as the Makuuchi criteria for resectable liver volume (8) and Barcelona Clinic Liver Cancer staging for hepatocellular carcinoma (9)]; (II) anatomical considerations (10) (to achieve oncologically satisfactory results or reduce postoperative ischemia/congestion); (III) intervention methods [ventral open or caudal laparoscopic approach (11)]; and (IV) available facilities (minimally invasive instruments, infrastructure facilities, and trained personnel). Advancements in the quality of medical imaging have enabled precise target localization. The optimal strategy can be usually formulated after considering every element of surgical planning, which will be an individualized surgical intervention that can minimize or completely prevent unnecessary or dangerous manipulations (12).
Through CTA, the cognitive tasks of liver resection can be divided into essential subtasks (exposure, tension creation, and target processing) that follow the basic surgical principles, which state that a surgical operation entails two components: imaging and manipulation (13). An artificially created tension (traction and counter-traction) can be delivered to the approximate site of intervention through open or laparoscopic approaches. The laparoscopic approach can create focal micro-tension and bypass the power transmitted through unnecessary or unrelated tissues. The subtasks can be delegated to each hand of the surgeon or to assistants, while the operator remains the main decision-maker during the operation.
Insight derived from comparison between open and laparoscopic approaches for liver resection: building a bridge connecting the basic surgical principle
Exposure, tension, and division: the basic surgical principle
The descriptive procedural steps of liver resection were performed as described previously (14). An analogical analysis between open and laparoscopic approaches (Figures 1,2) demonstrated the surgical principles, that is, exposure to make room for manipulation, and tension creation for division. This insight will help inexperienced surgeons to cope with surgical planning and decision-making intraoperatively and is discussed in the subsequent sections. Figure 3 presents the theoretical comparative advantages and disadvantages of open and laparoscopic liver resection procedures.
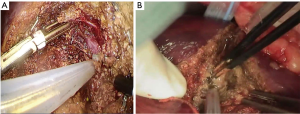
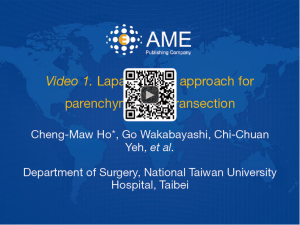
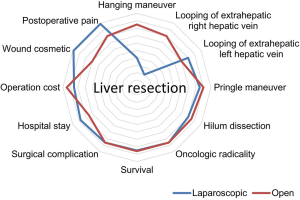
Exposure
Working plane determination and visual field construction
The basic element of surgical intervention is the determination of a visual field and working plane. Vision can be established through the naked eye or through cameras. The visual field is wide and most suitable for intervention when the observation is made perpendicular to the working plane, whereas and the visual field is pessimal if the plane of view is in parallel to the working plane (Figure 4A). An appropriate spatial position must be adjusted with respect to the working plane dynamically throughout the course of liver resection. Interventional instruments work most effectively and comfortably if placed on either side of the surgeon’s visual axis (Figure 4A). During laparoscopic manipulation, the camera lens should be set in a triangular pattern, with the operating arm lateral to and slightly above the endoscopic arm (16).
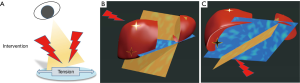
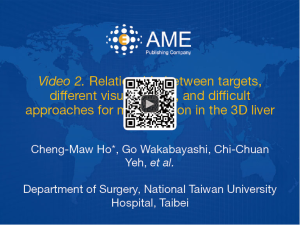
If the target lesion is located at the dome (Figure 4B) or at the dorsal and inferior part (Figure 4C) of the three-dimensional (3D) liver model, the working planes and visual fields are different, and therefore a customized approach must be adopted (Figure 5). An assistant’s role is to help the surgeon in ensuring that the surgery progresses smoothly because the visual field and working plane change along with the ongoing procedure. Every possible effort should be taken to turn the working plane toward a comfortable zone of visual field to ensure that the entire operation is performed smoothly. Otherwise, another (possibly more favorable) visual field with respect to the working plane might be required if the aforementioned effort is ineffective. For example, a posteriorly located liver lesion may be accessed from the dorsal side, and a lesion at the liver dorm may be accessed by creating a new visual field by opening the diaphragm from the thoracic cavity.
Detailed imaging of analytical anatomy facilitates the surgical planning of building the visual field
The surgical anatomy of the liver is crucial for surgical planning in a particular patient (18). The recognition of anatomical landmarks facilitates a smooth surgical procedure with relatively fewer potential complications (19,20). The 3D reconstruction of the vascular architecture in a specific patient allows the surgical team members to visualize the “invisible” aspects and makes them aware of the possible difficulties they may encounter within the liver during parenchymal transection (Figure 6A) (21,22). Compared with 2D computed tomography scans, 3D reconstruction leads to a considerably increased precision in tumor localization, even in inexperienced surgeons (23). Real-time 3D or virtual reality-assisted surgery will help surgeons perform liver resection safely in the near future (24-26).
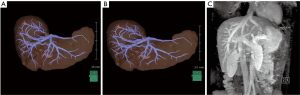
Moreover, the visual anatomical accommodation between neighboring hepatic venous vessels varies with different routes of visual direction, such as the caudal direction compared with the ventral side (Figure 6). Difficulty in vascular management using the caudal approach, as characterized through the laparoscopic method, may hinder surgeons from smoothly performing laparoscopic parenchymal transection. Therefore, a parenchyma transection approach that shifts from the cranial-caudal working plane (for open resection) to the ventro-dorsal working plane (for laparoscopic resection) should be emphasized. Familiarization with the laparoscopic anatomical orientation facilitates the transition of surgeons skilled at open liver resection to perform the laparoscopic procedure with less difficulty. Furthermore, the short distance (approximately 1 cm) between the middle hepatic vein and the hilum plate is a notable observation (Figure 6C). In addition, experts can swiftly adopt another “invisible” strategy at will intraoperatively, such as to create an alternative appropriate visual field in a narrow space to safely manage the hepatic veins (Figures 2,4B,C,7).
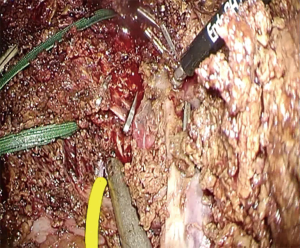
Whether parenchymal transection is initiated ventrally (open approach) or caudally (laparoscopic approach), the vision field is the most crucial aspect and it determines the utility degrees of hanging maneuver. The hanging maneuver is very useful in the open approach [particularly in minilaparotomy (27)] because it guides the transection line, shortens the visual field, and simultaneously creates tension on the back for transection (Figure 8A). The hanging maneuver is extremely difficult to perform using the laparoscopic approach and has fewer benefits when a laparoscopic approach with a caudal-cranial visual plane and ventro-dorsal working plane is considered. Nonetheless, it is readily feasible and can guide the direction of transection for experienced surgeons (Figures 8B,9).
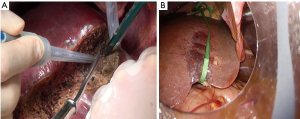
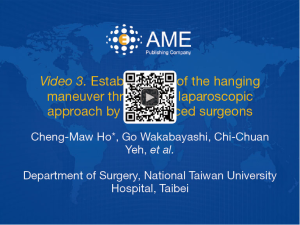
The looping of the extrahepatic hepatic veins before parenchymal transection on the right side (which can be easily accessible through the open approach; Figure 10A) is difficult through the laparoscopic approach compared to that on the left side (Figure 10B). Experienced surgeons may perform the looping by using intracostal trocars, thereby undoing the barrier (29). By contrast, the visualization and division of the short hepatic veins in front of the inferior vena cava (IVC) are more favorable using the caudal approach than the open ventral approach.
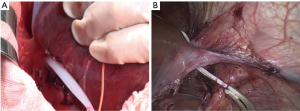
Tension creation at the working plane
Improved visual exposure can often simultaneously create adequate tension. For example, the mobilization of the right liver toward the left reveals the right posterior liver and also creates tension at the triangular ligament, facilitating further processing (such as ligament division). Gravity may assist in establishing visual exposure and tension creation by changing the patient’s position. Tension creation can be accomplished through a surgeon’s hands or instrument arms. Tension can be microspecific, and therefore can be minimally manipulated or may transmit the power through the gross surrounding mediator tissues. When tension is created in a gross-transmitting fashion, it may cause an extensive manipulation of nontargeted tissues and result in prolonged postoperative recovery phase and occasionally in morbidity. Therefore, targeted tension creation should be achieved during the intervention. Tension can be theoretically created in all dimensions; however, general surgeons are more accustomed to create it in the left-right axis. As described previously, a hanging maneuver creates tension from the back toward the front and simultaneously guides the direction of transection. Appropriate tension creation during the manipulation ensures that the cutting plane is straight and not zigzag.
Target manipulation
It is crucial to actively identify surgical histology and histopathology when target processing in liver resection. Unexpected large vessels or bile ducts exposed during the operation may be a hint, implying a review of the medical imaging correlation and reorientation of the spatial relationship between the working plane and visual field. Parenchyma splitting can be processed using energy devices [such as cavitron ultrasonic surgical aspirator (CUSA), harmonic scalpel, LigaSure, En-Seal, or electric coagulation], clamp-crushing, or a combination of several techniques depending on the surgeon’s preference and handling capability. Vascular or bleeding control can be accomplished by a near-site or proximal root approach (30).
Near-site vascular control includes either physical tie/clips/sutures or energy devices for sealing/coagulation (such as monopolar/bipolar coagulators, Tissue Link, En-Seal, or LigaSure). In addition, proximal root vascular inflow control using the Glissonian approach marks the demarcation line and guides the cutting plane. Moreover, bile duct processing requires additional attention because the complications of bile leak can be difficult and occasionally troublesome. The stump of a large bile duct after transection is more securely closed by (auto)sutures than clipping alone.
Overall, it is not surprising to realize that laparoscopic hepatic parenchymal transection varies greatly among experts (31,32). Selecting the most suitable technique and device or “multidisciplinary” combination of strategies for laparoscopic hepatic parenchymal transection can be challenging and should be tailored to each surgical scenario, for example, whether the location of the lesion is deep-seated from the liver surface and the surrounding anatomical territory will render the surgical planning different (33). Altogether, understanding the problems and strengths of each surgical approach can facilitate design of a versatile surgical solution or innovation that is most suitable for a particular scenario, such as two procedures in the same robotic session for a patient who requires cholecystectomy and prostatectomy (34).
Conclusions
In conclusion, the fundamental surgical principles for the establishment of a visual field to expose the target lesion and the creation of local tension at the working plane for liver resection are applicable for both the open and laparoscopic approaches, although this manifests in highly complex ways. The application of the rationales and understanding of the interaction between the surgical principles and other paramedical concerns (such as patient tolerability, available facilities, or the teamwork of trained personnel) could help trainee surgeons to visualize the “invisible” mind-sets of experienced surgeons and develop effective surgical strategies at will.
Acknowledgements
We thank Ms. Yi-Ling Lin and Department of Medical Education for providing technical support.
Footnote
Conflicts of Interest: The authors have no conflicts of interest to declare.
References
- Wakabayashi G, Cherqui D, Geller DA, et al. Recommendations for laparoscopic liver resection: a report from the second international consensus conference held in Morioka. Ann Surg 2015;261:619-29. [PubMed]
- Chiow AK, Lee SY, Chan CY, et al. Learning curve in laparoscopic liver surgery: a fellow’s perspective. Hepatobiliary Surg Nutr 2015;4:411-6. [PubMed]
- Ban D, Kudo A, Ito H, et al. The difficulty of laparoscopic liver resection. Updates Surg 2015;67:123-8. [Crossref] [PubMed]
- Osman H, Parikh J, Patel S, et al. Are general surgery residents adequately prepared for hepatopancreatobiliary fellowships? A questionnaire-based study. HPB (Oxford) 2015;17:265-71. [Crossref] [PubMed]
- Wingfield LR, Kulendran M, Chow A, et al. Cognitive task analysis: Bringing olympic athlete style training to surgical education. Surg Innov 2015;22:406-17. [Crossref] [PubMed]
- Sullivan ME, Ortega A, Wasserberg N, et al. Assessing the teaching of procedural skills: can cognitive task analysis add to our traditional teaching methods? Am J Surg 2008;195:20-3. [Crossref] [PubMed]
- Singh P, Aggarwal R, Tahir M, et al. A randomized controlled study to evaluate the role of video-based coaching in training laparoscopic skills. Ann Surg 2015;261:862-9. [Crossref] [PubMed]
- Makuuchi M, Kosuge T, Takayama T, et al. Surgery for small liver cancers. Semin Surg Oncol 1993;9:298-304. [Crossref] [PubMed]
- Bruix J, Sherman M, Llovet JM, et al. Clinical management of hepatocellular carcinoma. Conclusions of the Barcelona-2000 EASL conference. European Association for the Study of the Liver. J Hepatol 2001;35:421-30. [Crossref] [PubMed]
- Hasegawa K, Kokudo N, Imamura H, et al. Prognostic impact of anatomic resection for hepatocellular carcinoma. Ann Surg 2005;242:252-9. [Crossref] [PubMed]
- Wakabayashi G. Towards the 2nd International Consensus Conference on Laparoscopic Liver Resection. J Hepatobiliary Pancreat Sci 2014;21:721-2. [Crossref] [PubMed]
- Krawczyk M. Modern surgeon: Still a master of his trade or just an operator of medical equipment? Ann Surg 2017;266:703-5. [Crossref] [PubMed]
- Krummel TM. What is surgery? Semin Pediatr Surg 2006;15:237-41. [Crossref] [PubMed]
- Michalski CW, Billingsley KG. Modern technical approaches in resectional hepatic surgery. Surg Oncol Clin N Am 2015;24:57-72. [Crossref] [PubMed]
- Ho CM, Wakabayashi G, Yeh CC, et al. Laparoscopic approach for parenchymal liver transection. Asvide 2018;5:045. Available online: http://asvidett.amegroups.com/article/view/22572
- Berger R, Horgan S. Robotic-assisted bariatric surgery. In: Inabnet WB, DeMaria EJ, Ikramuddin S. editors. Laparoscopic bariatric surgery. Philadelphia: Lippincott Williams & Wilkins, 2005:309.
- Ho CM, Wakabayashi G, Yeh CC, et al. Relationship between targets, different visual angles, and difficult approaches for manipulation in the 3D liver model. Asvide 2018;5:046. Available online: http://asvidett.amegroups.com/article/view/22573
- Juza RM, Pauli EM. Clinical and surgical anatomy of the liver: a review for clinicians. Clin Anat 2014;27:764-9. [Crossref] [PubMed]
- Cho A, Okazumi S, Makino H, et al. Anterior fissure of the right liver--the third door of the liver. J Hepatobiliary Pancreat Surg 2004;11:390-6. [Crossref] [PubMed]
- Sugioka A, Kato Y, Tanahashi Y. Systematic extrahepatic Glissonean pedicle isolation for anatomical liver resection based on Laennec’s capsule: proposal of a novel comprehensive surgical anatomy of the liver. J Hepatobiliary Pancreat Sci 2017;24:17-23. [Crossref] [PubMed]
- Vohra S, Goyal N, Gupta S. Preoperative CT evaluation of potential donors in living donor liver transplantation. Indian J Radiol Imaging 2014;24:350-9. [Crossref] [PubMed]
- Soler L, Mutter D, Pessaux P, et al. Patient specific anatomy: the new area of anatomy based on computer science illustrated on liver. J Vis Surg 2015;1:21. [PubMed]
- Lamadé W, Glombitza G, Fischer L, et al. The impact of 3-dimensional reconstructions on operation planning in liver surgery. Arch Surg 2000;135:1256-61. [Crossref] [PubMed]
- Herfarth C, Lamadé W, Fischer L, et al. The effect of virtual reality and training on liver operation planning. Swiss Surg 2002;8:67-73. [Crossref] [PubMed]
- Soler L, Nicolau S, Pessaux P, et al. Real-time 3D image reconstruction guidance in liver resection surgery. Hepatobiliary Surg Nutr 2014;3:73-81. [PubMed]
- Olasky J, Sankaranarayanan G, Seymour NE, et al. Identifying opportunities for virtual reality simulation in surgical education: A review of the proceedings from the Innovation, Design, and Emerging Alliances in Surgery (IDEAS) Conference: VR Surgery. Surg Innov 2015;22:514-21. [Crossref] [PubMed]
- Nitta H, Sasaki A, Fujita T, et al. Laparoscopy-assisted major liver resections employing a hanging technique: the original procedure. Ann Surg 2010;251:450-3. [Crossref] [PubMed]
- Ho CM, Wakabayashi G, Yeh CC, et al. Establishment of the hanging maneuver through the laparoscopic approach by experienced surgeons. Asvide 2018;5:047. Available online: http://asvidett.amegroups.com/article/view/22574
- Gumbs AA, Gayet B. Adopting Gayet's techniques of totally laparoscopic liver surgery in the United States. Liver Cancer 2013;2:5-15. [Crossref] [PubMed]
- Tranchart H, O’Rourke N, Van Dam R, et al. Bleeding control during laparoscopic liver resection: a review of literature. J Hepatobiliary Pancreat Sci 2015;22:371-8. [Crossref] [PubMed]
- Otsuka Y, Kaneko H, Cleary SP, et al. What is the best technique in parenchymal transection in laparoscopic liver resection? Comprehensive review for the clinical question on the 2nd International Consensus Conference on Laparoscopic Liver Resection. J Hepatobiliary Pancreat Sci 2015;22:363-70. [Crossref] [PubMed]
- Toro JP, Patel AD, Lytle NW, et al. Detecting performance variance in complex surgical procedures: analysis of a step-wise technique for laparoscopic right hepatectomy. Am J Surg 2015;209:418-23. [Crossref] [PubMed]
- Otsuka Y, Kaneko H, Cleary SP, et al. What is the best technique in parenchymal transection in laparoscopic liver resection? Comprehensive review for the clinical question on the 2nd International Consensus Conference on Laparoscopic Liver Resection. J Hepatobiliary Pancreat Sci 2015;22:363-70. [Crossref] [PubMed]
- Akbulut Z, Canda AE, Cimen HI, et al. Two procedures at the same robotic session: robot-assisted laparoscopic radical prostatectomy and cholecystectomy. Surg Laparosc Endosc Percutan Tech 2011;21:e34-5. [Crossref] [PubMed]
Cite this article as: Ho CM, Wakabayashi G, Yeh CC, Hu RH, Sakaguchi T, Hasegawa Y, Takahara T, Nitta H, Sasaki A, Lee PH. Comprehensive evaluation of liver resection procedures: surgical mind development through cognitive task analysis. J Vis Surg 2018;4:21.